Exploring Supercells: Understanding Tornado and Storm Dynamics
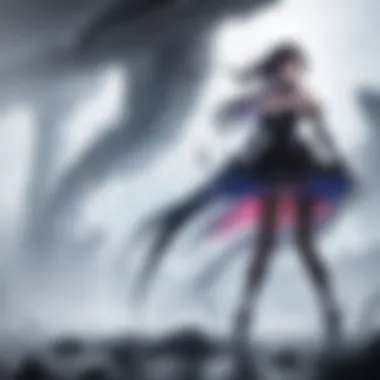
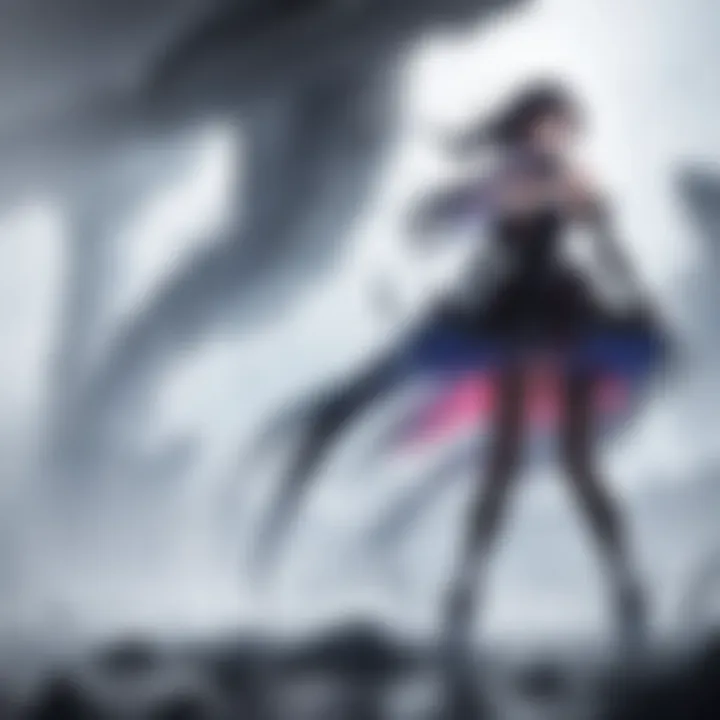
Intro
Supercells are complex storm systems that intrigue both meteorologists and weather enthusiasts. This article serves as an exposition of supercells, detailing their structure and the mechanisms that enable their formation. These storms are not only notable for their intensity but also for their unique characteristics that set them apart from typical thunderstorm systems.
Understanding supercells involves looking at their life cycle, meteorological conditions favorable for their development, and their potential to spawn tornadoes. By examining these factors, we aim to provide a thorough comprehension of how supercells function within the atmosphere and their relevance in severe weather forecasting.
This discourse lays the groundwork necessary for grasping the sometimes perilous phenomena associated with supercells. By the end, readers will have a clearer picture of what makes these storms a critical area of study in meteorology.
Prolusion to Supercells
The study of supercells is essential due to their unique structure and ability to generate severe weather phenomena such as tornadoes. Supercells are a specific type of thunderstorm characterized by a rotating updraft known as a mesocyclone. Understanding supercells provides key insights into storm dynamics, which is critical for accurate weather predictions. This knowledge not only aids meteorologists but also informs the public, enhancing safety during severe weather events.
Supercells differ from typical thunderstorms in several respects. They can last for hours and are capable of producing intense weather occurrences, including large hail, destructive winds, and, importantly, tornadoes. Studying these storms allows for improved forecasting and early warning systems, ultimately benefitting communities at risk of severe weather.
Defining Supercells
A supercell is defined primarily by its rotating nature. This rotation occurs within the updraft, creating a mesocyclone, which sets supercells apart from other storm types. They typically exhibit a well-defined structure, comprising three main components: the updraft, the downdraft, and the anvil shape at the top. The updraft is crucial, as it allows warm, moist air to rise and cool, fostering the development of severe weather.
Key features of supercells include their long lifespan and the potential to produce severe weather events. This characteristic distinguishes supercells from ordinary thunderstorms, which generally dissipate within a relatively short time frame. Moreover, the organization of supercells is precise; they often form in environments with specific atmospheric conditions conducive to rotation and intensity.
Historical Context
The recognition of supercells dates back to the mid-20th century, when meteorologists began to understand the fundamental processes behind severe weather systems. Initially, the study of thunderstorms was limited, but advances in radar technology improved monitoring capabilities and offered insights into storm dynamics.
Supercells have often been studied due to their association with significant tornado outbreaks, especially in the United States. The research led to the development of specific terminology and classification systems to distinguish supercells from other storm systems. Over the years, significant storms, such as the 1974 Super Outbreak, have underscored the power and destructiveness of supercells, prompting further investigation into their characteristics and formation.
As meteorological science evolved, so too did the understanding of supercells and their role in severe weather. Historical patterns, combined with ongoing research, contribute to the expertise required for effective forecasting and public safety measures. Through continuous study, meteorologists seek to unravel more complexities associated with these formidable storms.
Meteorological Characteristics of Supercells
Supercells are recognized for their distinct structure and behavior, making the study of their meteorological characteristics crucial for understanding severe weather phenomena. These storm systems present various attributes that determine their intensity and potential for destruction, especially regarding tornado generation. This section will focus on key features that characterize supercells, laying the groundwork for analyzing their impact on severe weather events.
Key Features
Updrafts
Updrafts are an essential element of supercells, as they are the upward-moving air currents that nourish these storms. The intensity and strength of an updraft can significantly influence a storm's development. A strong updraft can lead to more organized storm structures, capable of sustaining themselves for longer periods. One unique feature of updrafts is their ability to rotate, contributing to the formation of mesocyclones. This capability makes them a vital focus in studies related to supercell dynamics. However, they also present challenges, such as creating conditions favorable for hail formation, a serious hazard during severe storms.
Downdrafts
In contrast to updrafts, downdrafts represent the downward air movement within a storm. They play a critical role in the storm life cycle, particularly during the mature and dissipating stages. A key characteristic of downdrafts is their role in producing outflow boundaries—areas where cool air from the rain-cooled downdraft interacts with warm, moist surface air. This interaction can trigger new storms or enhance existing ones, thus exacerbating severe weather conditions. Although downdrafts contribute to storm dissipation, they can also bring damaging winds and contribute to flash flooding.
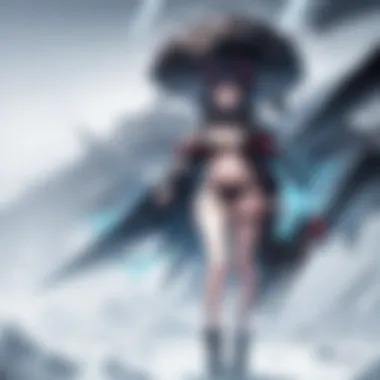
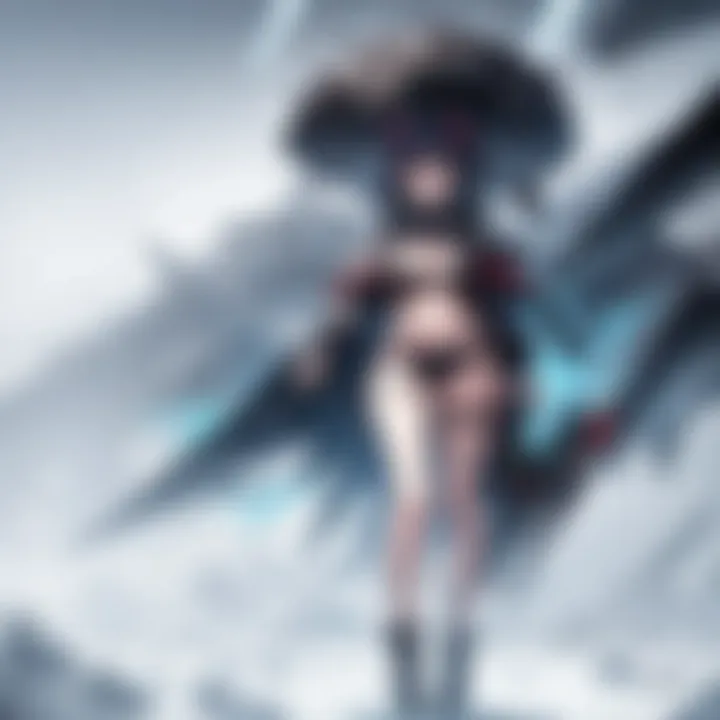
Mesocyclones
Mesocyclones are rotating updrafts commonly associated with supercells. They serve as a marker for severe weather potential, particularly tornado formation. The ability of a mesocyclone to maintain its structure over time is a key characteristic that illustrates the strength of a supercell. Mesocyclones can range in intensity, impacting the ability to predict severe weather. Their unique feature is the rotation at various altitudes, which can significantly increase the likelihood of tornadoes. Failure to accurately identify and assess mesocyclones can result in inadequate warnings for affected areas, highlighting the importance of this phenomenon in the context of supercell research.
Classification of Supercells
Understanding the classification of supercells is crucial for meteorological studies and accurate weather forecasting. This classification enables researchers to categorize these storms based on their structure and behavior, which in turn assists in predicting severe weather outcomes effectively.
Classical Supercells
Classical Supercells are characterized by their well-defined structure, including a strong updraft and a clear rotation. They are often associated with high precipitation rates and can produce large hail and significant tornadoes. The organization of this type of supercell makes it a popular choice for meteorologists, as its predictability allows for better warning systems. However, its intensity can lead to severe weather impacts, including flash flooding and wind damage.
HP Supercells
High-Precipitation (HP) Supercells differ from classical types in that they produce widespread rainfall and may obscure the visibility of tornadoes. They often present more complex dynamics due to the amount of moisture they carry, which can lead to rapid changes in behavior. The characteristic of overwhelming precipitation can make HP Supercells challenging for forecasters. The primary disadvantage lies in the difficulty of predicting tornadoes within these storms. Their potential for flash flooding is significant, making awareness crucial.
LP Supercells
Low-Precipitation (LP) Supercells are identified by their lower rainfall and hail production, but they can still generate strong winds and tornadoes. Their key characteristic is the ability to maintain a clear updraft, which enhances their tornado-producing potential. LP Supercells are of great interest due to their complex dynamics. They may cause less immediate risk from rainfall, but their tornadoes can be intense and often occur with less warning, presenting unique challenges for forecasting and public safety.
Formation of Supercells
Understanding the formation of supercells is crucial in comprehending their complex behavior and potential severe weather outcomes. Supercells arise from specific atmospheric conditions, which must converge to establish these formidable storm structures. This section highlights significant elements that contribute to supercell formation, examining the roles of instability, wind shear, and moisture. Each of these atmospheric components interacts to create the ideal environment for supercell development, making them key factors to consider.
Atmospheric Conditions Required
Instability
Instability is a primary factor in the formation of supercells. It refers to the atmosphere's ability to support vertical motion, crucial for the development of strong updrafts. When warm air near the surface rises and encounters cooler air aloft, it can create significant instability. This condition is beneficial because it allows storms to develop with greater intensity, thereby increasing the chances of severe weather, such as heavy rainfall and tornado formation. A unique feature of instability is its variability, which can change rapidly under different atmospheric situations. However, its disadvantage is that it is not always present, making its predictability challenging.
Wind Shear
Wind shear refers to the change in wind speed and direction with height. It is vital for supercell formation as it helps organize the storm structure. The presence of wind shear increases rotation within the storm, often leading to mesocyclone development—a hallmark of supercells. This characteristic is beneficial because it enhances the storm's rotational dynamics, aiding in the longevity and strength of the storm. Wind shear’s distinct feature is that it allows storms to tilt in the vertical plane, promoting sustained updrafts. However, too much shear can be detrimental, disrupting the storm's structure and leading to its dissipation.
Moisture
Moisture is another critical atmospheric condition needed for supercell formation. High humidity levels provide the necessary water vapor that condenses to form clouds and precipitation. This moisture is essential as it fuels the storm's energy. The key characteristic of moisture lies in its role in sustaining storm development; it enhances the updrafts and helps maintain turbulence within the storm system. A unique aspect of moisture is its impact on storm intensity and duration. If moisture is insufficient, the resulting storms tend to weaken quickly. Thus, an appropriate balance of moisture is required to cultivate a robust supercell.
Development Stages
The formation of supercells occurs through distinct development stages. Each stage is characterized by specific processes that are critical in shaping the storm's behavior.
Initiation
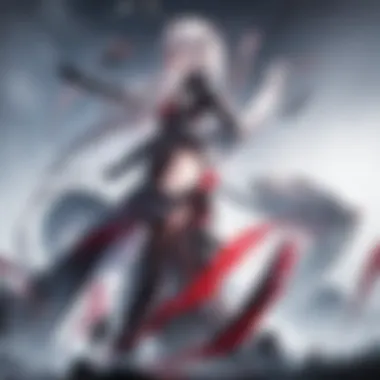
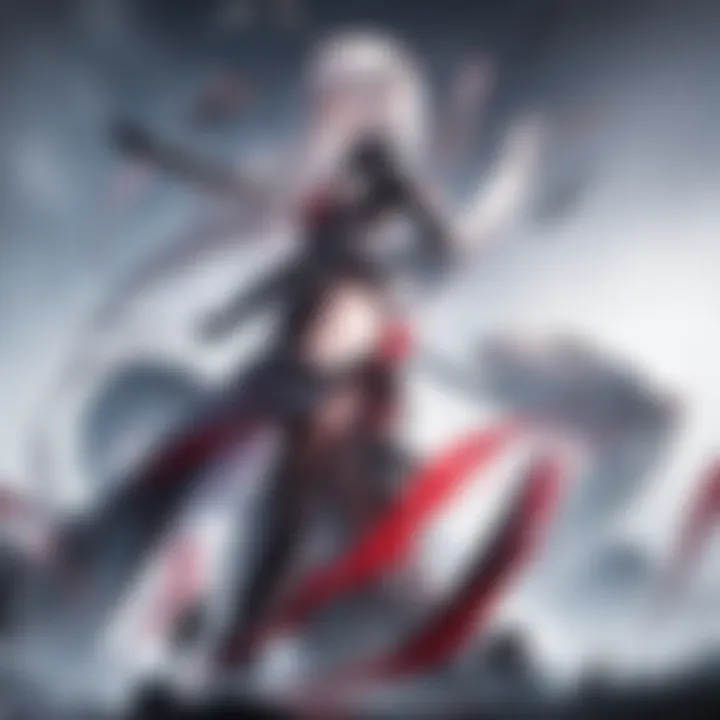
The initiation stage is the first step in the development of a supercell. It begins when local weather conditions combine to cause a rising parcel of warm, moist air. This stage is notable because it represents the moment the storm begins to form. The unique feature of this stage is that it often occurs in regions of low pressure, where lift mechanisms enable air to rise. However, the initiation phase can be unpredictable, making forecasting at this point challenging.
Organizing Stage
Following initiation, the storm enters the organizing stage. This phase is marked by the establishment of a well-defined updraft, where the storm starts to develop organized rotation. It is popular in this article because understanding this phase is essential for forecasting severe storms. The typical characteristic of this stage is the development of a mesocyclone. This organizing feature enhances the storm's structure and increases its potential for severe weather, but if conditions change, the storm can fail to organize effectively.
Mature Stage
The mature stage is the most intense part of a supercell's lifecycle. During this phase, the storm exhibits strong updrafts and downdrafts. It also produces significant precipitation, hail, and possibly tornadoes. This stage is critical because it represents the peak of the storm's intensity. A defining feature of the mature stage is the presence of a well-formed anvil cloud. The challenge during this phase is monitoring the development of severe weather events, which can occur rapidly and unexpectedly.
Dissipating Stage
The dissipating stage marks the transition of a supercell to its decay phase. The updraft weakens, and the storm begins to lose its structure. This stage is important as it significantly reduces the threat posed by the storm. The characteristic feature is the enfolding of the downdraft, which becomes dominant. While dissipating, the storm may still produce heavy rainfall or gusty winds, but the overall severity decreases. Recognizing this stage allows for more accurate forecasts and better preparedness for any lingering weather impacts.
Supercells and Tornadoes
The relationship between supercells and tornadoes is crucial for understanding severe weather events. Supercells are powerful storm systems capable of producing tornadoes. Recognizing their characteristics and the conditions that lead to tornado formation helps to enhance forecasting abilities. This section will explore how tornadoes originate from supercells, the fundamental conditions necessary for their development, and what makes this phenomenon significant.
Tornado Genesis in Supercells
Tornado genesis within supercells is a complex process that originates from specific storm dynamics. The core of a supercell features a rotating updraft called a mesocyclone. This rotation is essential for tornado formation. Once a mesocyclone is established, its intense updraft can stretch and intensify, leading to the development of a tornado.
For a tornado to form, the mesocyclone must interact with other environmental factors. This includes the stability of the atmosphere, wind shear, and humidity. The way these elements align can dictate whether a tornado will develop or dissipate quickly. Research shows that the stronger and better-organized a supercell, the higher the likelihood of tornado formation.
Conditions for Tornado Formation
Tornado formation is contingent upon various environmental conditions. Recognizing these can aid in predicting when and where tornadoes might strike:
- Instability: A warm, moist air mass needs to be present at the surface, while cooler air is found aloft, leading to strong vertical motions.
- Wind Shear: Variations in wind speed and direction with height create the necessary rotation within the storm.
- Moisture: High humidity contributes to cloud formation, essential for storm development.
In summary, supercells serve as the breeding ground for tornadoes, each aspect of their structure and the surrounding environment playing an integral role in the genesis of these destructive phenomena. Understanding these elements is vital for improving predictive models and enhancing public safety.
Impact of Supercells
Supercells represent a significant focus in meteorology due to their unique characteristics and their capacity to produce severe weather phenomena. Understanding the impact of supercells is essential not only for forecasting but also for enhancing public safety and preparedness. Supercells can lead to destructive tornadoes, damaging hail, and intense rainfall. These storms are rare, yet when they occur, their consequences can be catastrophic.
Severe Weather Events Associated with Supercells
Hail
Hail forms during supercell thunderstorms, primarily during the updraft phase of the storm. As water droplets are lifted into the atmosphere, they encounter freezing temperatures and freeze into balls of ice. This process occurs multiple times, with the stones gaining layers as they are cycled through the storm. Hail is notable for its potential to cause extensive damage to crops, vehicles, and buildings. Its size can vary significantly, from small pea-sized pellets to massive stones surpassing golf ball size.
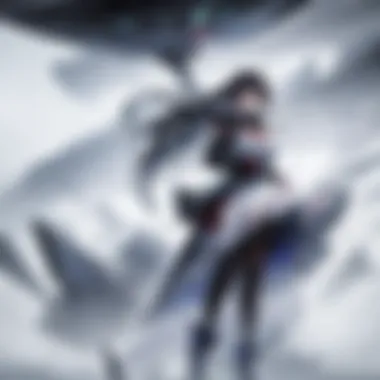
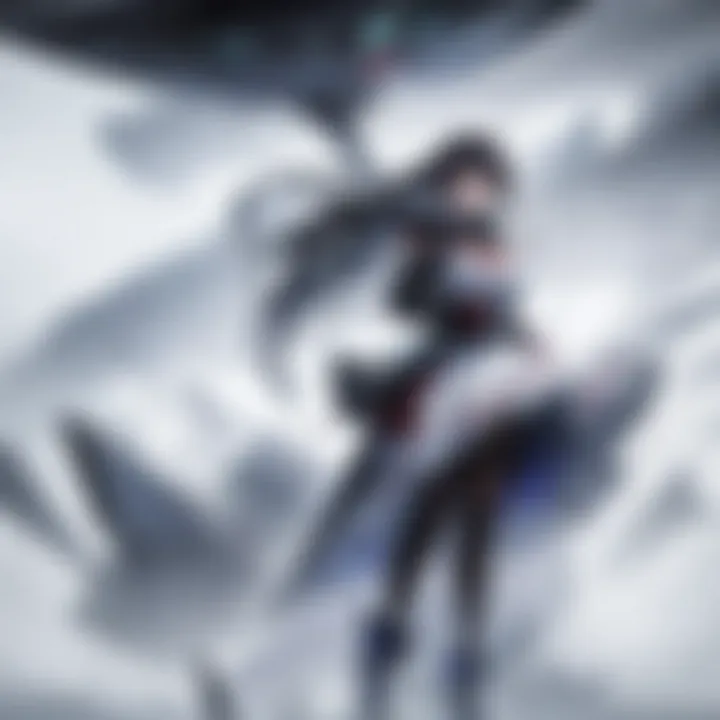
- Key Characteristic: The destructive capability of hail is particularly significant during severe storms.
- Contribution to Overall Topic: Understanding hail helps in assessing the potential risks associated with supercells.
- Unique Feature: Its formation is directly linked to the storm's strength. Stronger storms can produce larger hail, with severe storms leading to hailstones measuring over two inches.
- Advantages/Disadvantages: Recognizing hail's patterns can improve early warnings but also presents challenges for accurate forecasting.
High Winds
High winds are another key severe weather event typified by supercells. As the storm evolves, wind speeds can reach not just damaging levels but also become life-threatening. High winds occur both in the straight-line winds produced by downdrafts and in tornadoes. The damage they can inflict is vast, uprooting trees, damaging structures, and leading to extremely hazardous conditions on the road.
- Key Characteristic: The rapid onset of high winds can occur with little to no warning.
- Contribution to Overall Topic: High winds underscore the importance of timely updates in storm alerts.
- Unique Feature: Wind damage can be widespread, affecting regions well outside the immediate tornado path.
- Advantages/Disadvantages: High winds can provide indicators of storm severity, but their unpredictability poses challenges for emergency services.
Flash Flooding
Flash flooding is often a result of heavy rains produced by supercells. These storms can be particularly localized, leading to sudden surges of water in low-lying areas. Flash floods can occur within minutes or hours following intense rainfall, resulting in swift-moving water that can be very dangerous.
- Key Characteristic: Flash flooding is marked by its rapid onset and unpredictability.
- Contribution to Overall Topic: It highlights the need for comprehensive storm tracking and warning systems.
- Unique Feature: The localized nature of the flooding makes it difficult to predict where it will occur.
- Advantages/Disadvantages: Early detection systems can help mitigate risks, but the quick nature of flash floods can overwhelm communities that are unprepared.
Geographic Distribution
Supercells have a distinct distribution across the globe. The regions most affected are typically areas that experience favorable atmospheric conditions, including the United States' Great Plains and other similar climatic zones worldwide. Identifying where supercells frequently form aids in mounting effective storm response strategies and improving public awareness.
Supercell Research and Forecasting
Supercell research and forecasting is essential for understanding these complex storm systems. Supercells can lead to severe weather events that have significant impacts on communities. Gaining insights into these phenomena enhances safety and preparedness strategies. Advances in technology play a major role in this field. They help meteorologists identify potential supercell activity. This, in turn, can inform timely warnings and improve public safety measures.
Technological Advances
Radar Technology
Radar technology stands as a crucial tool in supercell research. It allows for real-time monitoring of storm development. One key characteristic is its ability to detect precipitation and storm rotation. This makes radar particularly beneficial for identifying the presence of supercells. Radar systems can provide detailed information on storm structure and behavior.
The unique feature of Doppler radar is its ability to measure wind speeds. This capability helps meteorologists assess the potential for tornado formation. However, there are limitations. Radar requires installation and maintenance, which can be costly. Additionally, its effectiveness can be hindered by terrain. Still, the advantages of rapid data acquisition and analysis make it invaluable.
Satellite Data
Satellite data complements radar technology. It provides a broader view of atmospheric conditions influencing supercells. A key characteristic of satellite imagery is its capacity to monitor cloud cover and thermal characteristics. This data is favorable for tracking storm systems over larger distances. It enables researchers to see developments that might not be captured by radar.
A unique feature of satellite data is its ability to observe storm systems from space. This perspective allows meteorologists to analyze regional weather patterns. However, there can be disadvantages. The temporal resolution may not be as high as radar, leading to potential gaps in data during rapid storm development. Nevertheless, satellite data enriches the overall understanding of supercells.
Future Directions in Research
Understanding Supercell Dynamics
Understanding supercell dynamics is vital for enhancing meteorological knowledge. Researchers aim to analyze the detailed processes behind supercell formation and behavior. A key characteristic is the focus on both thermal dynamics and wind patterns within supercells. This analysis is beneficial for improving forecasting accuracy. Insight into these processes can lead to better preparation for severe weather events.
The unique feature of this research approach is its multifaceted nature. It combines observational data with computer simulations. This integration provides deeper insights into the interactions that shape supercells. Yet, challenges remain. High variability in weather patterns can make generalization difficult. Nonetheless, progress in this area has the potential to refine our understanding significantly.
Improving Predictive Models
Improving predictive models is another crucial focus for supercell research. It involves refining the algorithms that meteorologists use for forecasting. A key characteristic of these models is their dependence on comprehensive and accurate input data. Enhanced models can provide timely alerts and improve response strategies during severe weather events.
The unique aspect of these predictive models is their ability to integrate various data sources. By combining radar, satellite, and ground observations, they can create a holistic picture of weather conditions. However, model accuracy can sometimes be inconsistent due to rapid changes in atmospheric conditions. Despite this, investing in model improvements is essential for enhancing public safety and preparedness.